Fillable Printable Draft Internal Memorandum
Fillable Printable Draft Internal Memorandum
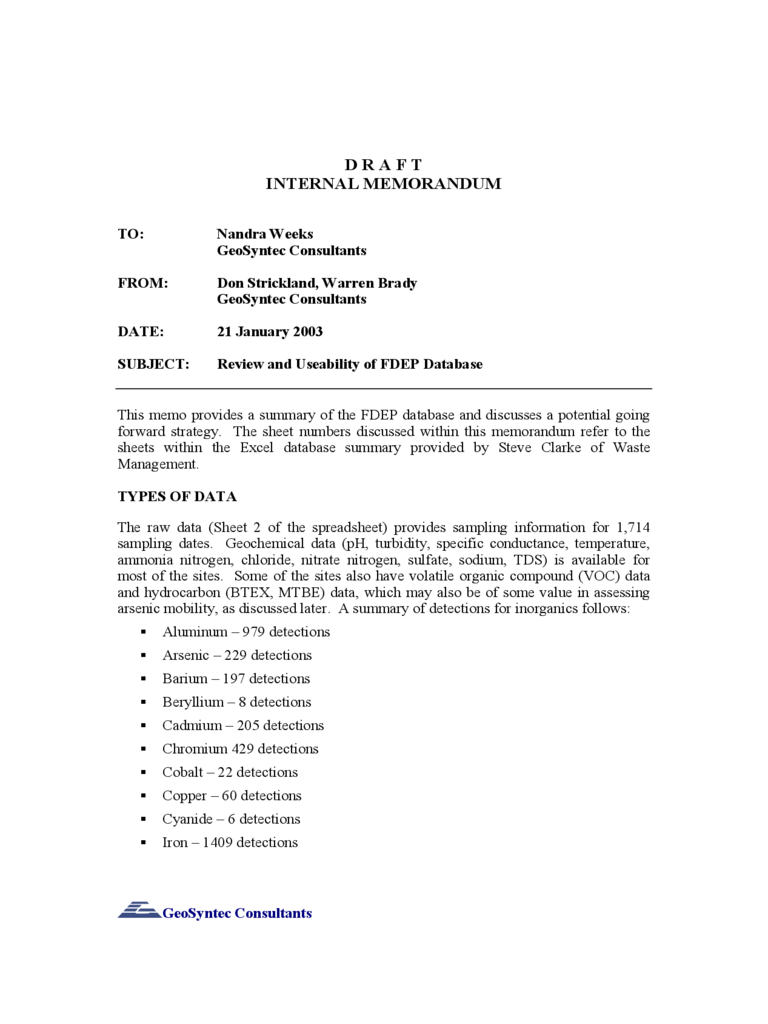
Draft Internal Memorandum
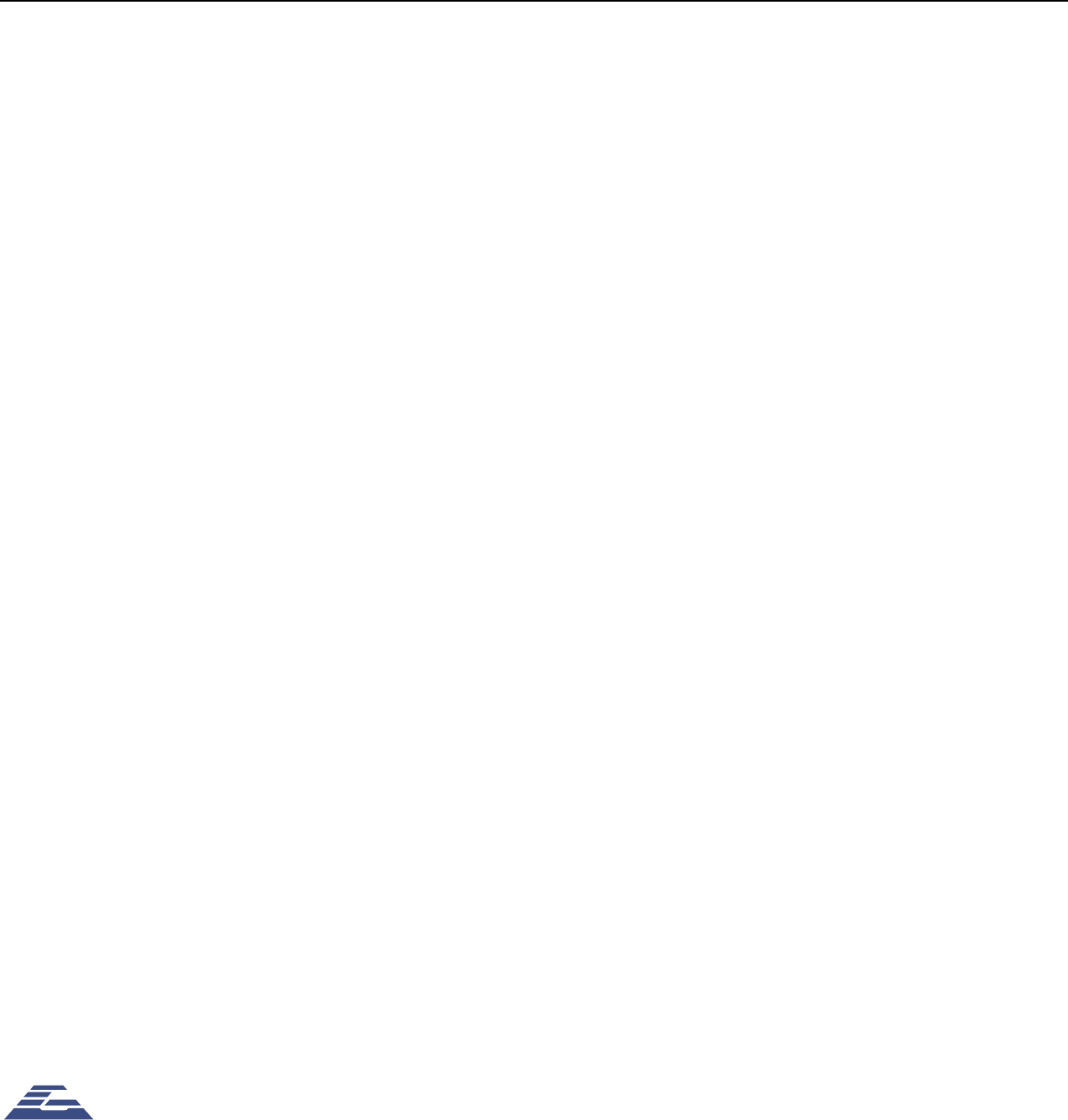
GeoSyntec Consultants
D R A F T
INTERNAL MEMORANDUM
TO: Nandra Weeks
GeoSyntec Consultants
FROM: Don Strickland, Warren Brady
GeoSyntec Consultants
DATE: 21 January 2003
SUBJECT: Review and Useability of FDEP Database
This memo provides a summary of the FDEP database and discusses a potential going
forward strategy. The sheet numbers discussed within this memorandum refer to the
sheets within the Excel database summary provided by Steve Clarke of Waste
Management.
TYPES OF DATA
The raw data (Sheet 2 of the spreadsheet) provides sampling information for 1,714
sampling dates. Geochemical data (pH, turbidity, specific conductance, temperature,
ammonia nitrogen, chloride, nitrate nitrogen, sulfate, sodium, TDS) is available for
most of the sites. Some of the sites also have volatile organic compound (VOC) data
and hydrocarbon (BTEX, MTBE) data, which may also be of some value in assessing
arsenic mobility, as discussed later. A summary of detections for inorganics follows:
Aluminum – 979 detections
Arsenic – 229 detections
Barium – 197 detections
Beryllium – 8 detections
Cadmium – 205 detections
Chromium 429 detections
Cobalt – 22 detections
Copper – 60 detections
Cyanide – 6 detections
Iron – 1409 detections
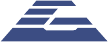
D R A F T
Internal Memorandum
21 January 2004
Page 2
GeoSyntec Consultants
Lead – 301 detections
Manganese – 109 detections
Mercury – 99 detections
Nickel – 45 detections
Selenium – 18 detections
Silver – 13 detections
Thallium – 15 detections
Vanadium – 59 detections
Zinc – 119 detections
Only 28 of the 1714 records in the raw data (Sheet 2) indicate that chromium was
present above the 100 µg/L threshold.
Sheet 1 (CCA hits in C&D Facilities based on University of Florida Data) evaluates
arsenic detections > 50 µg/L, chromium detections > 100 µg/L, and copper detections >
1000 µg/L at 79 sites. Most of this data comes from the time period of 1999 to 2001. It
is important to note that 47 of the 79 sites did not report copper.
DATA USEABILITY
As presented, the data set has some limitations. The raw data does not provide
detection limits; only “<” signs were reported for non-detected constituents.
Concurrently, the laboratory reporting limit may have been higher than the current or
proposed MCL. This would be more likely the case for older data, if applicable. The
database also does not clearly identify field duplicate samples making QA/QC
evaluation problematic. Definition of background also appears to be challenging in
some cases as presented. In one instance, as discussed later, the reported background
arsenic levels are higher than downgradient wells. The background issue may be one
example of human error. Other areas for error may include groundwater zone
demarcation, well screening, groundwater flow direction, well construction, etc.
Many of these limitations may be overcome by evaluating the specific reports related to
the sites to better answer some of these questions. It may also be useful to look at
turbidity and pH as indicators of sample quality. Poor monitoring well construction
and/or development often lead to turbidity problems. It should be noted that high
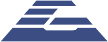
D R A F T
Internal Memorandum
21 January 2004
Page 3
GeoSyntec Consultants
turbidity is not necessarily indicative of poor construction and development; however, it
will certainly have an influence on metals concentrations.
ARSENIC EXCEEDANCE SUMMARY
Sheet 3 (Arsenic concentrations > 10 µg/L in UF and WACS databases) summarizes
sites with detections between 10 and 50 µg/L and greater than 50 µg/L. A total of 39
sites have at least one arsenic detection greater than 10 µg/L. While a total of seven
sites have reported arsenic concentrations greater than 50 µg/L. The sites with the
highest arsenic detections and most detections of arsenic higher than 50 µg/L include
the following:
Realco-Picketville;
Nine Mile Road; and
Country Road 672.
The WACS exceedance Summary (Sheet 4) identifies 13 facilities with one or more
exceedances of the 50 µg/L arsenic MCL and/or 100 µg/L chromium MCL.
SITE-SPECIFIC ASSESSMENT
Realco-Picketville (Sheet 5) compares arsenic concentrations over time in a
“background” and “compliance “well, and includes a time series graph. Both of these
wells, background included, have arsenic concentrations greater than 300 µg/L. In
many cases, the reported arsenic level in the background well is actually higher than the
compliance wells for most sampling events.
County Road 672 (Sheet 6) compares arsenic levels over time in ten wells. Most of the
problems (arsenic > 50 µg/L) are associated with a single well (MW-3), identified as
both a compliance and a detection well. Dade Recycling (Sheet 7) compares arsenic
levels in 17 wells over time. Nine Mile Road (Sheet 9) compares arsenic levels with
time in nine wells. There is one arsenic detection over 50 µg/L.
ARSENIC AND CHROMIUM CHEMISTRY
Arsenic and chromium groundwater solubility are going to be controlled by redox state,
and to a lesser extent pH. Arsenic is generally more soluble under reducing conditions,
while chromium generally more soluble under oxidizing conditions. Under reducing
conditions, chromium is reduced to the Cr 3+ species which, in most cases, will sorb
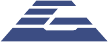
D R A F T
Internal Memorandum
21 January 2004
Page 4
GeoSyntec Consultants
strongly to the aquifer matrix. It is anticipated that leachate coming from a landfill
would be anaerobic and facilitate chrome reduction and sorption. This is consistent
with what is indicated in the database (only 27 exceedances in the database of 1714
records).
One of the major immobilization processes for arsenic is sorption to iron minerals like
ferri-hydrite that maintain a positive charge that captures negatively charged arsenic
anions. If the arsenic is coming out of the landfill, reducing conditions caused by
plentiful nutrients and a carbon source within the landfill may result in ferri-hydrite
reduction and subsequent arsenic release. This process may increase the solubility of
both naturally-occurring and arsenic emanating from the landfill. Arsenic may also be
reduced to the more soluble As +3 form that is charge-less. Other hypothesis and/or
scenarios for elevated arsenic and chromium levels in groundwater may include:
Arsenic and chromium were never really bound in the landfill and the aquifer
system does not have sufficient attenuation capacity to sorb arsenic and
chromium out of solution.
Reducing landfill leachate is reducing the attenuation capacity of the aquifer
system as it dissolves out iron minerals as it leaves the facilities.
Arsenic may also form insoluble complexes with sulfide. For this arsenic to ever re-
solubilize, the landfill leachate would have to be aerobic, which is highly unlikely.
CHARACTERIZING ARSENIC MOBILITY WITH GEOCHEMICAL DATA
We may be able to use available pH data and some of the geochemical parameters to
evaluate, to the extent possible, geochemical conditions conducive for arsenic and/or
chromium to be soluble in groundwater. Examples include:
DO – where available, information provides insights to the redox state.
Iron – may not be ferrous analysis, but if it is present in groundwater it is likely
to have a significant ferrous component. The same is true for manganese.
Nitrate and sulfate – more information that may be used to target the
groundwater redox in the absence of ORP measurements. Sulfate may also be
used to tell us if naturally occurring arsenic is being solubilized (probably not
with landfill leachate – as discussed previously).
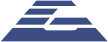
D R A F T
Internal Memorandum
21 January 2004
Page 5
GeoSyntec Consultants
Where present we can look at VOC data to see if the indigenous microorganisms
have a steady diet of a carbon source (drives groundwater reducing, which may
solubilize arsenic).
Ammonia – nutrients for indigenous microorganisms.
MODELING APPROACHES TO EVALUATE ARSENIC IMPACTS TO
GROUNDWATER
There are several modeling strategies that may assist in evaluating arsenic impacts to
groundwater. The key to proper application of modeling techniques is to make sure that
the modeling approach is appropriate to address the specific concern that is being
raised. In this case, there are at least three questions that the committee may be
interested in addressing:
• Is arsenic leaching from C&D landfills into groundwater?
• If so, are there significant groundwater impacts from arsenic leaching?
• Will any arsenic that leaches be sufficiently mobile to impact groundwater at a
compliance boundary?
It is likely that each of these questions would be addressed with a different type of
model. It is our understanding that the issue of arsenic leaching from landfills has been
studied in some detail by Dr. Townsend and Dr. Solo-Gabrielle of the Florida Center for
Solid and Hazardous Waste Research, and that much of this research is on-going. The
data collected in these studies are likely to be superior to any model-generated
predictions on the quantity and quality of landfill leachate emanating from C&D
landfills in Florida. Nonetheless, models for leachate quantity (HELP, SMLRM,
DMLRM and others) and quality (HELPQ, VLEACH and others) are available if
needed.
The second question would need to be addressed by using a combination of
geochemical equilibrium models (MINTEQ, PHREEQEC and others) and calculations
to evaluate the amount of arsenic that could be sorbed (primarily) by the aquifer matrix.
Arsenate (V) and arsenite (III) can both exist under normal groundwater conditions,
however, arsenate is more common. Arsenate (V) species tend to form complexes with
ferri-hydrites, as well as sorb to mineral complexes within the aquifer matrix. Arsenite
(III) will dominate in natural waters having lower Eh and pH, and will tend to be
substantially more mobile. A combination of groundwater sampling, aquifer matrix
sampling, and geochemical modeling can estimate the capacity of the aquifer to
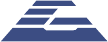
D R A F T
Internal Memorandum
21 January 2004
Page 6
GeoSyntec Consultants
assimilate arsenic (through sorption and complexation) and therefore predict whether
arsenic that leaches from a landfill would have significant impacts to groundwater.
The compliance boundary question can be addressed using any number of solutions that
can be categorized as “Kd-approach” models. Most commonly used transport models
and analytical solutions would fall into this category. They are relatively simple to set
up and can estimate a “rate” of arsenic movement (for a fixed source input) by
estimating a site-specific distribution coefficient (Kd). Many of these models can also
predict a maximum concentration for a particular point in time or space. They do not
address the “assimilative capacity” issue that was discussed in the previous paragraph.
Moreover, most assume a linear sorption isotherm which does not limit the amount of
solute that can be sorbed by the aquifer matrix.
RECOMMENDATIONS
Following further evaluation of the data by the Groundwater Subcommittee and
collection of additional information from FDEP for specific landfill sites, we
recommend that the CCA TAG define their objectives (specifically, which of the three
questions above need to be answered and/or are there others that need to be answered).
Once the objectives are defined, an appropriate model(s) can be developed to answer
the specific issues of concern.